The Eichrom DGA Resins are extraction chromatographic materials containing either N,N,N’,N’-tetra-n-octyldiglycolamide (DGA Resin, Normal) or N,N,N’,N’-tetra-2-ethylhexyldiglycolamide (DGA Resin, Branched). The structure of the DGA molecule is shown in Figure 1 (R = 1-octyl or 2-ethyl-1-hexyl). The DGA Resins have a bed density of 0.39 g/mL and a functional capacity of 7-8 mg Eu per mL of resin. (1)
Data for the extraction of selected metal ions by TRU, DGA, Normal and DGA, Branched resins is shown in Figures 2 and 3. The DGA Resins compliment or provide an alternative to TRU Resin in many applications due to their high affinity for trivalent rare earths and actinides, such as Y(III), Lu(III), Tb(III), Am(III), Cm(III), and Ac(III). Compared to TRU resin, the DGA resins exhibit higher retention of trivalent rare earths and actinides, lower retention of U(VI), less sensitivity to competition from Fe(III), and higher competition from Ca(II).
Trivalent actinides and rare earths are strongly retained on the DGA resins from HNO3, while Fe(III), Na(I), K(I), Mg(II) and many other common matrix ions are rejected. Trivalent actinides and rare earths can be recovered from the DGA Resins with dilute HCl. Light rare earths may also be stripped from the DGA, Branched using dilute (0.01-0.05M) HNO3. Th may also be recovered from DGA, Branched with dilute HCl (0.05 -0.25 M), but efficient recovery of Th from DGA, Normal requires the addition of HF (0.10M HCl – 0.05M HF or 3M HNO3 – 0.25M HF) or oxalic acid (0.10M ammonium bioxalate). Metal ions typically have lower retention (Dw or k’) on DGA, Branched compared to DGA, Normal.
DGA, Normal resin has been used extensively in the purification of 225Ac and 227Ac for nuclear medicine applications. (2,3) The DGA, Normal resin is particularly effective for concentrating Ac from HNO3 solutions, converting Ac solutions from HNO3 to HCl, and removing key metal ion impurities, such as Th, Fe, Ca, Ba, Ra and La. Figure 4 shows the acid dependency of k’ for Ac(III), La(III) and selected divalent metal ions in nitric acid and hydrochloric acid on DGA, Normal. In HNO3 media, Ra shows no real affinity for the resin, while the Ac k’ is >1000 for 1-3M HNO3. Ac can be stripped from DGA, Normal with 0.05-2.0M HCl. Using 2.0M HCl to recover Ac can provide additional decontamination from Th isotopes, which will remain on DGA, Normal in 2M HCl.
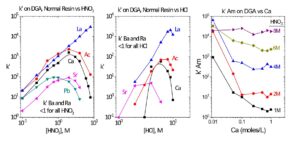
Figure 4. k’ La, Ac, Ca, Sr, Ba, Ra, and Pb on DGA, Normal resin vs HNO3 and HCl. k’ Am on DGA, Normal resin vs Ca and HNO3.
The k’ for Ac decreases above 2-3M HNO3, while the k’ for rare earth cations continues to remain >1000. This provides a mechanism for the separation of Ac and rare earths. Ac can be eluted with 8-10M HNO3, while the rare earths remain on the DGA Resin. (2)
Sr, Pb, and Ca show moderate uptake on DGA, Normal from ~0.5-4 M HNO3, while Be, Mg, Ba, and Ra exhibit essentially no retention. Samples containing high Ca content should be loaded onto DGA Resin from 8M HNO3 to keep the k’ value for Ca low and limit the impact of Ca on the retention of other metal ions. The influence of Ca and HNO3 on the k’ for Am(III) is shown in the right panel of Figure 4. From 1-3M HNO3, where Ca uptake is greatest, high concentrations of Ca dramatically reduce the retention of Am on the DGA, Normal. From 4-8M HNO3, where Ca uptake decreases, Ca concentrations of up to 1.0 mole/L have very little impact on Am retention. The impact of Ca is pronounced, even for Am(III) which is strongly retained by DGA, Normal. For metal ions retained less strongly, such as La(III), Ac(III), and U(VI), the impact of Ca is even more severe.
In Figure 5, the uptake of Sr(II) and Y(III) on DGA, Normal from both nitric acid and hydrochloric acid is presented. Y(III) is much more strongly retained on the resin than Sr(II) from both acids across all concentrations. Coupling the Eichrom Sr Resin with DGA-Normal enables excellent separation of Y from Sr for radiopharmaceutical purification (90Y or 86Y) or for rapid 89/90Sr measurement procedures. (4-6) The Y/Sr separation is equally effective for high specific activity 90Sr (Figure 6) and bulk stable Sr targets (Figure 7). (1) For large masses of Sr, 6-8M HNO3 or HCl can be used to further decrease Sr retention on DGA, Normal and improved Y recovery. DGA, Normal resin has also been proposed for the separation of Sr from complex matrices, such as raw acidified urine. (7) The DGA, Normal resin is less sensitive to Na(I) and K(I) than Sr Resin, so recovery of Sr can be higher with the DGA, Normal resin when these metal ions are present at high concentrations. The DGA, Normal resin, however, is not as selective for Sr as Sr Resin, so additional steps may be needed to remove metal ions such as Pb(II), Ca(II), and U(VI) which could co-elute with Sr(II) from DGA, Normal. (8)
Figure 8 shows the uptake of various transition and post transition elements on DGA, Normal. (1) Bismuth is retained from 0.05-8M HNO3 and HCl and will remain on the DGA, Normal when recovering metal ions in dilute HCl (0.05-0.25M). Bi will elute from DGA, Normal when recovering metal ions with oxalic acid or ammonium bioxalate. When decontamination from Bi is critical to the analysis, Bi may be removed from DGA, Normal resin with 9-10M HCl, before recovering dilute HCl, oxalic acid, or ammonium bioxalate. (9)
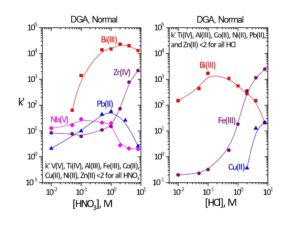
Figure 8. k’ for select transition and post transition metal ions on DGA, Normal resin vs HNO3 and HCl.
Iron is strongly retained from higher concentrations of HCl, but shows little uptake from nitric acid. Fe(III), Bi(III), Ga(III) and other metal ions that form anionic chloride complexes can also lead to an enhancement in the extraction of metal ions by DGA resins. (10,11) This enhancement is thought to arise from the replacement of Cl– counter ions with the larger anionic metal-chloride species (for example [FeCl4]–), Figure 9. (12) The enhancement observed with Al is not due to anionic aluminum chloride, but simply additional chloride provided AlCl3.
The DGA Resins are uniquely suited for the separation of trivalent actinides and lanthanides from a wide range of metal ion impurities. (13,14) Separation factors for adjacent rare earths are typically higher on DGA, Normal, while metal ion uptakes on the DGA, Branched are typically lower, allowing recovery of metal ions such as Y(III) in smaller volumes of dilute HCl (Figure 10) and recovery of light rare earth metal ions and Ac in dilute HNO3. In addition to analytical applications, the DGA Resins have been used in nuclear medicine for the purification of 44Sc, 47Sc, 86Y, 90Y, 225Ac, 227Ac, 161Tb, and 177Lu.
Note: High specific activity 90Y may also be recovered from DGA, Normal with 0.05 – 0.10M ammonium bioxalate. When mg-mg amounts of stable Y or other rare earths are present, recovery of Y with bioxalate can be poor due to the precipitation of rare earth oxalates in the resin bed.
DGA, Normal resin has been used in conjunction with the LN series of resins for the separation of adjacent rare earth metal ions for nuclear medicine applications, such as 177Lu from Yb targets and 161Tb from Gd targets. (13,15) Irradiated rare earth metal or oxide targets are dissolved in nitric or hydrochloric acid, and the acid concentration reduced through evaporation or concentration of the rare earths on DGA resin. Primary separations of the adjacent rare earth metal ions are then performed using either LN or LN2 resin.
Separation factors (α) for the adjacent rare earths are not high (1.6 < α < 5.0). Therefore, several large columns (20-50 mL) of LN or LN2 resin on fine particle size support (25-50 μm) operated at elevated temperature may be required to achieve the desired purity of the final product. The DGA, Normal resin is utilized between successive separations on LN or LN2 to concentrate the rare earths, convert the mobile phase from a relatively high concentration of HNO3 to a dilute HCl appropriate for loading LN/LN2, while also removing key metal ion impurities, such as Ca and Fe.
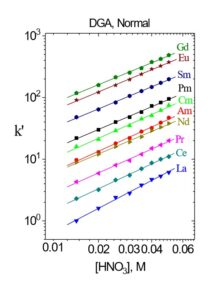
Figure 12. k’ for select trivalent rare earth and actinide metal ions on DGA, Normal resin from HNO3.
Values of k’ for selected trivalent rare earths and actinides are presented in Figures 11 and 12, while separation factors and typical elution curves for rare earths on DGA, Normal and DGA, Branched are presented in Table 2 and Figures 13-14, respectively. Metal ion retention and separation factors for adjacent rare earths are typically higher for DGA, Normal than DGA, Branched. Also, separation factors are relatively high for the light rare earths (La-Tb) on the DGA resins, but decrease substantially for the higher rare earths (Dy-Lu). Sc(III) elutes near Dy on the DGA resins, while Y elutes near Tb (DGA, Normal) or Gd (DGA, Branched). Due to the poor separation factors for heavy rare earths on the DGA resins, the LN series of resins is typically used to perform the primary separation of adjacent heavy rare earths, while DGA is used to concentrate the rare earths between successive LN series columns.
For the lighter rare earths, some separation from adjacent rare earths and trivalent actinides may be achieved using the DGA resins. For example, the relatively large separation factor for Am(III) over La(III) on DGA, Normal facilitates the removal of La(III) from the Am/Cm fraction when a LaF3 precipitate is used to preconcentrate actinides and remove matrix from fused soil, building materials, or other silicate containing or difficult matrices. La(III) removal is achieved by rinsing the DGA, Normal resin with 3M HCl (Figure 15). Rinsing with lower concentrations of HCl results in significant loss of Am(III). Some removal of other light rare earths is also achieved with the 3M HCl rinse, however, removal of heavier rare earths from the Am/Cm fraction requires methods such as the TEVA-thiocyanate separation. (16)
DGA, Normal resin may also be used in the analysis of 210Po from difficult matrices. From simple aqueous matrices, 210Po can be autodeposited on metal discs and measured directly by alpha spectrometry. However, in more complex aqueous samples and solid matrices, direct autodeposition of Po may not be effective. Po is retained strongly from HCl and HNO3 by DGA, Normal and can be recovered in dilute HNO3 (Figure 16) providing a means to separate Po from many common matrix ions and potentially interfering alpha emitters. (9) Once separated on DGA, Normal, Po can be autodeposited onto metal planchets or collected via BiPO4 microprecipitation onto Resolve™ filters prior to measurement by alpha spectrometry.
References
1) Horwitz E.P., McAlister D.R., Bond A.H., Barrans R.E., Novel Extraction Chromatographic Resins Based on Tetraalkyldiglycolamides: Characterization and Potential Applications, Solvent Extraction Ion Exch., 23, 219 (2005). (HP104)
2) Mastren, T., Radchenko, V., Owens, A., Copping, R., Boll, R., Griswold, J.R., Mirzadeh, S., Wyant, L.E., Brugh, M., Engle, J.W., Nortier, F.M., Birnbaum, E.R, John, K.D., Fassbender, M.E. 2017. Simultaneous Separation of Actinium and Radium Isotopes from a Proton Irradiated Thorium Matrix. Nature Scientific Reports, 7, 8216. doi:10.1038/s41598-017-08506-9.
3) D.R. McAlister, E.P. Horwitz, “Selective Separation of Radium and Actinium from Bulk Thorium Target Material on Strong Acid Cation Exchange Resin from Sulfate Media,” Applied Radiation and Isotopes, 140, 18-23 (2018).
4) Eichrom Application Note AN-1615, “90Y Generator”
5) Eichrom Application Note AN-1623, “Separation of 86Y From Sr Target”
6) Eichrom Application Note AN-1624, “Options for 89Sr and 90Sr Determination”
7) R.L. Jones, O. Piraner, G. Xaio, “How to Eliminate the Need for a Vacuum Box Pre-Analytical System,” The 64th Radiobioassay and Radiochemical Measurements Conference, Santa Fe, NM, October 27 – November 1, 2019.
8) D.R. McAlister, E. Rush, D. Silvestri, E.P. Horwitz, “Extraction of Selected Metal Ions with Mixtures of N,N,N’,N’-tetra-n-octyldiglycolamide and 4,4′(5′)-di-t-butylcyclohexano 18-crown-6,” Solv. Extr. Ion Exch., in press (2020).
9) S.L. Maxwell, D.R. McAlister, R. Suldowe, “Novel Rapid Oxidizing Fusion Method to Determine Polonium-210 in Air Filters,” Applied Radiation and Isotopes, 153, (2019):108833. https://doi.org/10.1016/j.apradiso.2019.108833
10) D.R. McAlister and E.P. Horwitz, “Synergistic enhancement of the extraction of trivalent lanthanides and actinides by tetra(n-octyl)diglycolamide from chloride media,” Solv. Extr. Ion Exch., 26(1), 12-24 (2008).
11) D.R. McAlister and E.P. Horwitz, “Synergistic extraction of trivalent lanthanides and actinides from acidic chloride media by tetraalkyldiglycolamides,” Proceedings of the 2008 International Solvent Extraction Conference, Canadian Institute of Mining, Metallurgy and Petroleum, pp. 1099-1104, (2008).
12) M.A. Antonio, D.R. McAlister, E.P. Horwitz, “Europium(III) Diglycolamide Complex: Insights into the Coordination Chemistry of Lanthanides in Solvent Extraction,” Dalton Transactions, 44(2), 515-521 (2015).
13) E. P. Horwitz, D. R. McAlister, A. H. Bond, R. E. Barrans, J. M. Williamson, “A Process for the Separation of 177Lu from Neutron Irradiated 176Yb Targets,” Applied Radiation and Isotopes, 63, 23-36 (2005).
14) S. Tachimori, S. Susuki, Y. Sasaki, A. Apichaibukol, “Solvent Extraction of Alkaline Earth Metal Ions by Diglycolic Amides from Nitric Acid Solutions,” Solv. Extr. Ion Exch., 21(5), 707-715 (2003).
15) A. Dash, M.R.A Pillai, F.F. Knapp, Jr. “Production of 177Lu for Targeted Radionuclide Therapy: Available Options,” Nucl. Med. Mol. Imaging, 49(2), 85-107 (2015).
16) Eichrom Application Note AN-1806, “Actinide/Rare Earth Separation (TEVA-SCN).